- Journal List
- Toxicol Res
- v.30(1); 2014 Mar
- PMC4007045

Studies on the Toxicity and Distribution of Indium Compounds According to Particle Size in Sprague-Dawley Rats

Abstract
Objectives: The use of indium compounds, especially those of small size, for the production of semiconductors, liquid-crystal panels, etc., has increased recently. However, the role of particle size or the chemical composition of indium compounds in their toxicity and distribution in the body has not been sufficiently investigated. Therefore, the aim of this study was to examine the effects of particle size and the chemical composition of indium compounds on their toxicity and distribution. Methods: Male Sprague-Dawley rats were exposed to two different-sized indium oxides (average particle sizes under 4,000 nm [IO_4000] and 100 nm [IO_100]) and one nano-sized indium-tin oxide (ITO; average particle size less than 50 nm) by inhalation for 6 hr daily, 5 days per week, for 4 weeks at approximately 1 mg/m3 of indium by mass concentration. Results: We observed differences in lung weights and histopathological findings, differential cell counts, and cell damage indicators in the bronchoalveolar lavage fluid between the normal control group and IO- or ITO-exposed groups. However, only ITO affected respiratory functions in exposed rats. Overall, the toxicity of ITO was much higher than that of IOs; the toxicity of IO_4000 was higher than that of IO_100. A 4-week recovery period was not sufficient to alleviate the toxic effects of IO and ITO exposure. Inhaled indium was mainly deposited in the lungs. ITO in the lungs was removed more slowly than IOs; IO_4000 was removed faster than IO_100. IOs were not distributed to other organs (i.e., the brain, liver, and spleen), whereas ITO was. Concentrations of indium in the blood and organ tissues were higher at 4 weeks after exposure. Conclusions: The effect of particle size on the toxicity of indium compounds was not clear, whereas chemical composition clearly affected toxicity; ITO showed much higher toxicity than that of IO.
INTRODUCTION
Indium was first used in large quantities as indium phosphide for the manufacture of semiconductors in the 1980s. More recently, indium-tin oxide (ITO) has been widely used as a thin film material for liquid crystal displays, which accounts for 87% of the total indium demand. As of 2009, 70 tons of indium was produced, 215 tons was imported, and 543.6 tons was used in Japan. It is estimated that approximately 960 tons of ITO was used in Korea (1).
In 1961, Leach et al. (2) exposed rats to 24~97 mg/m3 (approximately 12.5~50 mg/m3 of indium; mass median aerodynamic diameter of 0.5 μm) indium oxide (IO) for 4 hr a day for 3 months. They observed a wide range of symptoms that were similar to alveolar edema, and the presence of alveolar protein, which lasted for 12 weeks after exposure. The American Conference of Governmental Industrial Hygienists (ACGIH) quoted this report when determining the threshold exposure value (TLV) for indium in 1969 (3). The same value that was recommended as the TLV by the ACGIH, 0.1 mg/m3, is used as an exposure limit for indium and its compounds in Korea (4).
The interest in controlling indium and its compounds has increased in recent years owing to several reports of mortality following severe lung injury in Japan. Indium and its compounds were handled without any special restrictions in Japan until 2001, when the death of an indium-handling worker was reported. Following this accident, the Ministry of Health, Labour and Welfare (MHLW) in Japan required a material safety data sheet for indium, set a permeable concentration of 0.1 mg/m3 for indium compounds, and stated that workplace environment monitoring must be conducted every 6 months (5). In addition, the MHLW amended the permeable concentration for indium and its compounds to 3 × 10–4 mg/m3 after Nagano’s report, which described the carcinogenicity of ITO; the target concentration was determined to be 0.01 mg/m3 owing to technical and economic constraints (6). ITO was designated as a material requiring special management because of its carcinogenicity (7-9).
Primary particle size or surface area is important for evaluating the hazards of particulate matters (10), because smaller particles, which have a large gross surface area, are more toxic. Therefore, the United Sates’ National Institute for Occupational Safety and Health proposed exposure limits for nanomaterials on the basis of their surface area (11).
Currently, indium is produced in the form of nanomaterials that are smaller than 100 nm in many workplaces, and ITO smaller than 20 nm has been identified in the workplace environment (12). Therefore, particle size must be considered when evaluating the hazards of indium and its compounds. However, many previous toxicological studies were performed using larger particles. Thus, additional toxicological studies should be conducted to assess the hazards of small-sized indium compounds.
This study was conducted to provide toxicity information on indium compounds according to particle sizes and chemical compositions that are likely to exist in the workplace.
MATERIALS AND METHODS
Particles. Indium (III) oxide (Samchun chemicals 10640, Seoul, Korea; IO-4000), indium (III) oxide (Aldrich 632317, St. Louis, MO, USA; IO_100), and ITO (Aldrich 544876, St. Louis, MO, USA; ITO) were chosen for the study owing to their planned particle size and chemical composition.
Animals. Five-week-old male specific pathogen-free Sprague-Dawley (SD) rats were obtained from Central Lab Animal Inc. (Seoul, Korea). The rats were exposed to IOs and ITO after a 2-week acclimation period. During the experimental period, the rats were housed in a room with controlled temperature (23 ± 2℃), humidity (55 ± 7%), and a 12-hr light/dark cycle. The rats were fed with filtered water and a rodent diet (LabDiet 5053, PMI Nutrition, St. Louis, MO, USA) ad libitum. Pulmonary function was measured both at the end of exposure for 4 weeks and 4 weeks after exposure using non-invasive, unrestrained plethysmography (Buxco PLY 3123, Wilmington, NC, USA). All animal studies were approved by the animal ethics committee to ensure appropriate animal care for research.
Exposure to IOs. IOs were dispersed in distilled water and sonicated at a total power of 1,170 Joule by using a probe-type ultrasonicator (Vibra Cell VC-750, Newtown, CT, USA), and particle sizes were measured using Zetasizer (Nano Z 590, Malvern Instruments, Malvern, UK) to ensure that the suspensions were well dispersed. Then, the suspensions of IOs and ITO were aerosolized at 8 L of airflow per minute through a 0.8-mm-diameter venturi nozzle in a nose-only inhalation chamber (NITC System, HCT, Incheon, Korea). The total airflow was adjusted to 20 L per minute to meet the chamber’s ventilation rate of 15 times per hour. The concentrations of indium in the inhalation chambers were measured using inductively coupled plasma mass spectrometry (ICP-MS; Agilent Technologies 7500CE, Santa Clara, CA, USA) after collection on a 37-mm nitrocellulose membrane filter (Millipore GSWP, 0.22 μm, County Cork, Ireland) at a rate of 1 L per minute. The size distribution of IOs was analyzed using an optical particle counter (GRIMM Technologies Inc. model 1.108, Douglasville, GA, USA) and their morphological shapes were identified under a transmission electron microscope (Hitachi H7100FA, Tokyo, Japan). Rats were exposed to aerosolized indium compounds 6 hr a day, 5 days per week, for 4 weeks.
Bronchoalveolar lavage. Rats were anesthetized with isoflurane (Ilsung Pharmaceuticals Co., Seoul, Korea) and the tracheae were cannulated. Then, the lungs were lavaged 5 times with 3 mL of calcium- and magnesium-free phosphate buffered saline (PBS, pH 7.4), and the lavage fluids were centrifuged at 1,500 rpm for 10 min (Hanil Union 32R, Incheon, Korea). The supernatants were stored at –80℃ until protein and lactate dehydrogenase (LDH) assays were performed, and the total number of precipitated cells was counted using a Coulter Counter (Drew Science, Hemavet 950, Miami Lakes, FL, USA). The cells in the lavage sample were then centrifuged using Cyto centrifuge (Hanil Cellspin, Incheon, Korea). The centrifuged cells were then stained with Diff-Quick staining solution (Sysmex, Kobe, Japan), and the numbers of macrophages, lymphocytes, and polymorphonuclear leukocytes (PMNs) were measured by counting approximately 300 cells under a microscope at 20× magnification. LDH and albumin levels in the lavage fluid were measured using a biochemistry analyzer (Toshiba TBA 20FR, Tokyo, Japan).
Histopathology. Lungs were fixed in 10% neutral buffered formalin solution and embedded in paraffin. After staining with hematoxylin and eosin or Masson’s trichrome, the lung samples were examined by light microscopy at 100× magnification.
Measurement of indium levels in the blood and tissues. Well-dried lung, liver, spleen, and brain tissues were digested in a 10-fold volume of 65% nitric acid (Merck, Whitehouse Station, NJ, USA), and the levels of indium in the lungs, liver, spleen, and brain were measured using ICP-MS (Agilent technologies 7500CE, Santa Clara, CA, USA). Indium levels in the blood and serum were measured after the samples were diluted 10 times with 65% nitric acid.
Statistical analysis. Results are presented as the mean ± standard deviation. Data were analyzed using ANOVA, followed by a post hoc analysis based on the t-test or Mann-Whitney rank sum test to determine differences between groups. Statistical analyses were performed using Sigma-Plot 12 (Systat Software Inc., San Jose, CA, USA). Differences were considered significant when the P value was < 0.05.
RESULTS
Identification of indium compounds. The dynamic light scattering diameters of IO_4000, IO_100, and ITO_50 were 757.3 ± 105.5 nm, 435.9 ± 37.4 nm, and 176.2 ± 2.2 nm, respectively (Table 1). TEM revealed the following findings: IO_4000 showed the least degree of agglomeration; ITO_50 showed the highest degree of agglomeration; and IO_100 showed a medium degree in agglomeration (Fig. 1).
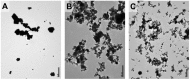
Aerosolization of indium compounds. The mass median aerodynamic diameters of the aerosols in the nose-only inhalation chamber were 1.09 μm for IO_4000, 0.66 μm for IO_100, and 0.17 μm for ITO_50, as measured using an optical particle counter (OPC). The median aerodynamic diameters were 0.43 μm for IO_4000, 0.42 μm for IO_100, and 0.13 μm for ITO_50 (Fig. 2). Indium concentrations in the inhalation chamber averaged 1.30 ± 0.14 mg/m3 for IO_1000, 1.13 ± 0.33 mg/m3 for IO_100, and 1.13 ± 0.33 mg/m3 for ITO_50, as measured using ICP-MS (Table 2).
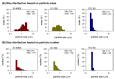
Clinical observations. During the exposure and postexposure periods, the body weights and clinical symptoms of the groups exposed to indium compounds were not significantly different from those of the normal control group (data not shown). Groups exposed to IO_4000 or IO_100 showed no statistically different respiratory function, whereas there were significant changes in respiratory frequency, tidal volume, and minute volume in the groups exposed to ITO_50 for 4 weeks; these symptoms persisted for 4 weeks after exposure (Table 3). Regarding hematological effects, there were no significant changes in white blood cell counts in any of the groups after the 4-week exposure period, although the counts increased at 4 weeks after the exposure period (data not shown).
Effects on cells in the bronchoalveolar lavage fluid. The total number of cells in bronchoalveolar lavage (BAL) fluid was 7.6 times higher in IO_4000-exposed rats, 3.1 times higher in IO_100-exposed rats, and 28.8 times higher in ITO_50-exposed rats than in the normal control group. Moreover, the ITO_50 group had a higher total number of cells compared to the IO_4000 group and IO_100 group (Table 4). This observation was likely due to an increased number of PMNs and macrophages. In the 4-week exposure experiment, PMNs accounted for 57.4% of the total cell number in the IO_4000 group, 57.9% in the IO_100 group and 86.9% in the ITO_50 group, while PMNs were rarely found in the normal control group. A similar pattern was observed at 4 weeks after exposure. The levels of albumin and LDH in the BAL fluids revealed a similar pattern as was noted with BAL cell differentiation in the IO_50 groups. In the 4-week exposure experiment, the concentrations of albumin and LDH were 28.0 times and 28.8 times higher, respectively, in the IO_50 group compared to the corresponding concentrations in the normal control group, and persisted for 4 weeks after exposure. Thus, the cellular damage caused by IO_4000 and IO_100 was lesser than that caused by ITO_50 (Table 5).
Histopathological effects. On average, the lungs of the IO_4000- and IO_100-exposed rats weighed 22% and 12% more, respectively, than the lungs of the rats in the normal control group. Notably, the average lung weight of ITO_50-exposed rats was 2.2 times higher than that of the rats in the normal control group (Table 6). Histopathological analyses in the ITO_50-exposed rats revealed alveolar proteinosis, migration of alveolar macrophages, alveolar wall fibrosis, type II epithelial cell hyperplasia/hypertrophy, perivascular inflammation, and pleural thickening with prominent mesothelial cells. These observations were also noted in the IO_4000- and IO_100-exposed rats, although the severity was much lesser than that in the ITO_50-exposed rats. The symptoms in the lungs were observed at both 4-week exposure and 4 weeks after the exposure period, and alveolar proteinosis and alveolar wall fibrosis were more severe at 4 weeks after the exposure period. IO_4000 and IO_100 caused a similar level of lung damage. No symptoms attributable to indium exposure were found in other organs (Table 7 and Fig. 3).
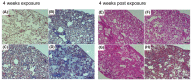
Biodistribution of indium. Next, indium inhaled through the nose-only inhalation chamber was assessed in the lungs. The amount of indium deposited in the lungs increased in the following order: IO_4000 > IO_100 > ITO_50. This particular order was likely due to the different average concentrations of indium (1.30 ± 0.41 mg/m3 for IO_4000, 1.13 ± 0.33 mg/m3 for IO_100, and 1.13 ± 0.33 mg/m3 for ITO_50). The distribution and elimination characteristics of IOs and ITO in the body were different. Indium was rapidly eliminated from the lungs and was not distributed to other organs, such as the brain, liver, and spleen, in the IO_4000-and IO_100-exposed groups. In contrast, indium was eliminated slowly from the lungs and detected in other organs in the ITO-exposed group. Indium migrated to different organs in the following order: spleen > liver > brain. Except for the lungs, the concentrations of indium increased at 4 weeks after exposure in the other organs. The concentrations of indium in the IO_100-exposed group decreased more slowly than that in the IO_4000-exposed group, but the pattern of distribution and elimination in other the organs were similar. Furthermore, the concentrations of indium in the ITO_50-exposed group were much lower than the current biological indicator suggested by the Japan Society for Occupational Health (0.176 μg/L at 4-week exposure and 0.265 μg/L at 4 weeks after the exposure) (Fig. 4).
DISCUSSION
This study was inspired by the fact that the use of indium compounds is increasing, and indium compounds are typically produced in the form of nanomaterials, whose toxico-logical properties have not been identified.
Several background points apply to this study. First, the use of indium compounds is growing, which increase the concern for workers’ health; the amount of indium used to produce semiconductors, displays, rechargeable batteries, and other high-tech gadgets is significantly increasing, and it is becoming a threat to the health of workers, especially those who deal with indium compounds. New indium-related diseases, such as “indium lung,” have been documented, and these diseases have resulted in deaths in Japan, the United States, and China (13-18). Second, there is great concern regarding the use of nanomaterials whose toxic properties have not been sufficiently investigated. Even with the widely accepted assumption that smaller particles (i.e., nanomaterials) are more toxic, appropriate remains questionable (19). Lastly, most of toxicological data from hazard assessments to date were generated using larger particles, although indium compounds (especially indium-tin oxide) are produced at the nano-scale (2,20-24). Therefore, this study was performed to investigate the toxicity and distribution of indium compounds according to their particle size and chemical composition to fill the knowledge gap concerning the hazards of indium compounds. To this end, 2 different particle sizes of IOs (i.e., IO_4000, whose primary particle size is 4 μm or less and IO_100, whose primary particle size is 100 nm or less) and ITO (whose primary particle size is 50 nm or less) were selected as experimental materials. Indium is mostly used as an ITO target for the production of liquid crystal displays. ITO target is typically a mixture of 90% of IO and 10% tin oxide, and it is sintered at a high temperature of 1500℃ (25). Although sintered ITO is likely to have different toxicological properties, the metal alloy, which is often considered to be merely a mixture of metals, has not been studied from the alloy-toxicity perspective (26). Recent occupational accidents suggest the need to investigate the toxicological effects of sintered alloys. Therefore, we raised 4 questions to bridge the knowledge gap: 1) Are data sufficient for hazard assessment? 2) Are there sufficient scientific grounds supporting biological indicators? 3) Can the lung damage caused by indium compounds be reverted? and 4) Should indium compounds be controlled differently depending on their individual particle size or chemical composition?
The indium compounds used in this study agglomerated easily in distilled water. The agglomerate sizes of IO_4000, IO_100, and ITO_50 in aqueous solution were 757.3 μg, 435.9 μg, and 176.2 μg, respectively, and the sizes of aerosolized indium compounds generated in the nose-only inhalation chamber averaged 1.09 μg for IO_4000, 0.66 μg for IO_100, and 0.17 μg for ITO_50.
After exposure for 4 weeks, the average concentrations of indium were 1.30 ± 0.41 mg/m3 in the IO_4000-exposed group, 1.13 ± 0.33 mg/m3 in the IO_100-exposed group, and 1.09 ± 0.37 mg/m3 in the ITO_50-exposed group. Although the exposure to IO_4000 was 15% greater than that to IO_100 and 19% greater than that to ITO_50, it was allowable. Neither body weight changes nor clinical symptoms were observed after the exposure and post-exposure periods in any of the experimental groups. However, typical injury symptoms, such as effects on respiratory function, inflammatory responses in the alveoli, and histopathological changes in the lungs, were clearly observed after exposure to indium compounds. The results of this study indicate that the chemical composition of indium compounds had a greater influence on respiratory function than individual particle size; IO_100 and IO_4000 did not affect respiratory function, whereas ITO_50 significantly affected respiratory function. The total number of cells in the lavage fluids increased 7.6-fold in response to IO_4000 exposure, 3.1-fold in response to IO_100 exposure, and 28.8-fold in response to ITO_50 exposure compared to the findings in the normal controls. PMNs accounted for 57.4% of the cells observed after IO_4000 exposure, 57.9% of the cells observed after IO_100 exposure, and 86.9% of the cells observed after ITO_50 exposure, whereas there were no PMNs in the normal controls. The effects were clear in both the IO- and ITO-exposed groups, but the severity was much higher in the ITO-exposed group. The LDH and albumin levels in the BAL fluid, which is a typical indicator of cell damage, showed a pattern similar to that observed in the case of cell differentiation: levels of both LDH and albumin increased slightly in the BAL fluid in the IO_4000- and IO_100-exposed groups and increased markedly in the BAL fluid in the ITO_50 exposed group.
A 4-week recovery period was not sufficient to mitigate the toxic effects of IO_4000, IO_100, and ITO. Effects on respiratory function, alveolar inflammation, and lung injuries persisted after the 4-week recovery period. White blood cell counts increased over the course of the recovery period. Interestingly, we observed a different distribution of indium in the body depending on the particle size and chemical composition, which in turn influenced the toxic effects. IO_4000 and IO-100 were quickly removed from the lungs and were not distributed to the brain, liver, and spleen; IO_4000 was removed from the lungs at a faster rate than IO_100. In contrast, ITO_50 was not eliminated rapidly, and during the 4-week recovery period, it distributed to other organs in the following order: spleen > liver > brain. The concentration of indium in the blood and other tissues increased over the 4-week recovery period. The concentrations of indium in the blood (4-week exposure: 0.176 μg/L; 4 weeks after exposure: 0.265 μg/L) were much lower than 3 μg/L, which is considered a biological indicator by the Japan Society for Occupational Health (JSOH). Thus, indium may induce lung damage at concentrations below the level of the current Japanese biological indicator (27).
The results of this study suggest that indium compounds of different chemical composition should be controlled differenly; i.e., indium-tin oxide is much more toxic than indium oxide.
References
Formats:
- Article |
- PubReader |
- ePub (beta) |
- PDF (2.3M) |
- Citation
- Pulmonary toxicity in mice by 2- and 13-week inhalation
exposures to indium-tin oxide and indium oxide aerosols.[J Occup Health. 2011]
Nagano K, Nishizawa T, Eitaki Y, Ohnishi M, Noguchi T, Arito H, Fukushima S. J Occup Health. 2011; 53(3):234-9. Epub 2011 Mar 16.
- Toxicology and carcinogenesis studies of indium
phosphide (CAS No. 22398-90-7) in F344/N rats and B6C3F1 mice (inhalation
studies).[Natl Toxicol Program Tech Rep ...]
National Toxicology Program.. Natl Toxicol Program Tech Rep Ser. 2001 Jul; (499):7-340.
- Pulmonary toxicity of indium-tin oxide production
facility particles in rats.[J Appl Toxicol.
2016]
Badding MA, Fix NR, Orandle MS, Barger MW, Dunnick KM, Cummings KJ, Leonard SS. J Appl Toxicol. 2016 Apr; 36(4):618-26. Epub 2015 Oct 15.
- The toxicology of indium tin oxide.[Environ Toxicol Pharmacol. 2016]
Bomhard EM. Environ Toxicol Pharmacol. 2016 Jul; 45:282-94. Epub 2016 Jun 10.
- The toxicology of indium oxide.[Environ Toxicol Pharmacol. 2018]
Bomhard EM. Environ Toxicol Pharmacol. 2018 Mar; 58:250-258. Epub 2018 Feb 7.
- Comparing plasma, serum and whole blood indium
concentrations from workers at an indium-tin oxide (ITO) production
facility[Occupational and environmental...]
Harvey RR, Virji MA, Edwards NT, Cummings KJ. Occupational and environmental medicine. 2016 Jul 25; 73(12): 864-867
- Application of the ICRP respiratory tract model to
estimate pulmonary retention of industrially sampled indium-containing
dusts[Inhalation toxicology. 2017]
Stefaniak AB, Virji MA, Badding MA, Cummings KJ. Inhalation toxicology. 2017 Jun 8; 29(4): 169-178
- Comparative toxicity and biodistribution of copper
nanoparticles and cupric ions in rats[International
Journal of Nanom...]
Lee IC, Ko JW, Park SH, Lim JO, Shin IS, Moon C, Kim SH, Heo JD, Kim JC. International Journal of Nanomedicine. 2016 Jun 16; 11: 2883-2900
- Pulmonary toxicity of indium-tin oxide production
facility particles in rats[Journal of applied
toxicology ...]
Badding MA, Fix NR, Orandle MS, Barger MW, Dunnick KM, Cummings KJ, Leonard SS. Journal of applied toxicology : JAT. 2015 Oct 15; 36(4): 618-626
- PubMed
PubMedPubMed citations for these articles
- Studies on the Toxicity and Distribution of
Indium Compounds According to Partic...
Studies on the Toxicity and Distribution of Indium Compounds According to Particle Size in Sprague-Dawley RatsToxicological Research. 2014 Mar; 30(1)55
- Cell-Phone Addiction: A Review
Cell-Phone Addiction: A ReviewFrontiers in Psychiatry. 2016; 7()
- Noninvasive human brain stimulation.
Noninvasive human brain stimulation.Annu Rev Biomed Eng. 2007;9:527-65.PubMed
- Non-invasive Human Brain Stimulation in
Cognitive Neuroscience: A Primer.
Non-invasive Human Brain Stimulation in Cognitive Neuroscience: A Primer.Neuron. 2015 Sep 2;87(5):932-45. doi: 10.1016/j.neuron.2015.07.032.PubMed
- Electromagnetic Energy Radiated from Mobile
Phone Alters Electrocardiographic Re...
Electromagnetic Energy Radiated from Mobile Phone Alters Electrocardiographic Records of Patients with Ischemic Heart DiseaseAnnals of Medical and Health Sciences Research. Jul-Dec 2012; 2(2)146
Your browsing activity is empty.
Activity recording is turned off.
See more...- The
inhalation toxicity of indium sesquioxide in the rat.[ORINS Rep US At Energy Comm. 1961]
LEACH LJ, SCOTT JK, ARMSTRONG RD, STEADMAN LT, MAYNARD EAORINS Rep US At Energy Comm. 1961 May 10; UR-590():1-30.
- In
search of the most relevant parameter for quantifying lung inflammatory
response to nanoparticle exposure: particle number, surface area, or
what?[Environ Health Perspect. 2007]
Wittmaack KEnviron Health Perspect. 2007 Feb; 115(2):187-94.
- Interstitial
pneumonia developed in a worker dealing with particles containing indium-tin
oxide.[J Occup Health. 2003]
Homma T, Ueno T, Sekizawa K, Tanaka A, Hirata MJ Occup Health. 2003 May; 45(3):137-9.
- Pulmonary
alveolar proteinosis in an indium-processing worker.[Chin Med J (Engl). 2010]
Xiao YL, Cai HR, Wang YH, Meng FQ, Zhang DPChin Med J (Engl). 2010 May 20; 123(10):1347-50.
- Review The new toxicology of sophisticated materials:
nanotoxicology and beyond.[Toxicol Sci.
2011]
Maynard AD, Warheit DB, Philbert MAToxicol Sci. 2011 Mar; 120 Suppl 1():S109-29.
- The
inhalation toxicity of indium sesquioxide in the rat.[ORINS Rep US At Energy Comm. 1961]
LEACH LJ, SCOTT JK, ARMSTRONG RD, STEADMAN LT, MAYNARD EAORINS Rep US At Energy Comm. 1961 May 10; UR-590():1-30.
- Oral
toxicity of indium in rats: single and 28-day repeated administration
studies.[J Occup Health. 2008]
Asakura K, Satoh H, Chiba M, Okamoto M, Serizawa K, Nakano M, Omae KJ Occup Health. 2008; 50(6):471-9.
- Inhalation
carcinogenicity and chronic toxicity of indium-tin oxide in rats and
mice.[J Occup Health. 2011]
Nagano K, Nishizawa T, Umeda Y, Kasai T, Noguchi T, Gotoh K, Ikawa N, Eitaki Y, Kawasumi Y, Yamauchi T, Arito H, Fukushima SJ Occup Health. 2011; 53(3):175-87.